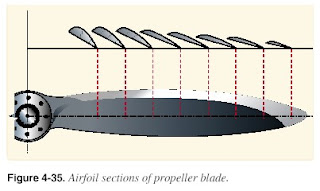
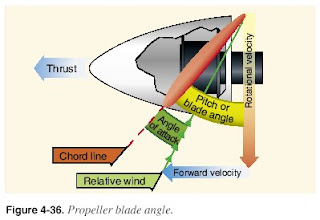
The pitch of a propeller may be designated in inches. A propeller designated as a “74-48” would be 74 inches in length and have an effective pitch of 48 inches. The pitch is the distance in inches, which the propeller would screw through the air in one revolution if there were no slippage.
When specifying a fixed-pitch propeller for a new type of aircraft, the manufacturer usually selects one with a pitch that operates efficiently at the expected cruising speed of the aircraft. Every fixed-pitch propeller must be a compromise because it can be efficient at only a given combination of airspeed and revolutions per minute (rpm). Pilots cannot change this combination in flight.
When the aircraft is at rest on the ground with the engine operating, or moving slowly at the beginning of takeoff, the propeller efficiency is very low because the propeller is with sufficient speed to permit reach their full efficiency. In this blade is turning through the air at relatively little thrust for the amount it.
Of a propeller, consider first its rotational and forward. As shown by forces in Figure 4-36, each section of downward and forward. The angle wind) strikes the propeller blade is produced by this angle causes the engine side of the propeller blade to pressure, thus creating thrust.
Also creates thrust because it is shape of a wing. As the air flows pressure on one side is less than that reaction force is produced in the pressure. The airflow over the wing force (lift) is upward. In the case mounted in a vertical instead of a decreased pressure is in front of (thrust) is in a forward direction. the result of the propeller shape also in terms of the mass of air these terms, thrust equals mass by slipstream velocity minus The power expended in producing air mass movement. On average, approximately 80 percent of the torque (total horsepower absorbed by the propeller). The other 20 percent is lost in friction and slippage. For any speed of rotation, the horsepower absorbed by the propeller balances the horsepower delivered by the engine. For any single revolution of the propeller, the amount of air handled depends on the blade angle, which determines how big a “bite” of air the propeller takes. Thus, the blade angle is an excellent means of adjusting the load on the propeller to control the engine rpm.
The blade angle is also an excellent method of adjusting the AOA of the propeller. On constant-speed propellers, the blade angle must be adjusted to provide the most efficient AOA at all engine and aircraft speeds. Lift versus drag curves, which are drawn for propellers, as well as wings, indicate that the most efficient AOA is small, varying from +2° to +4°. The actual blade angle necessary to maintain this small AOA varies with the forward speed of the aircraft.
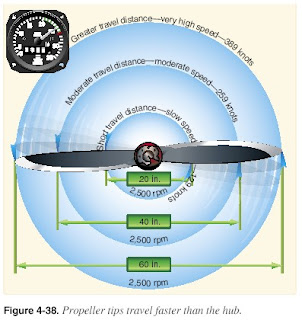
Usually 1° to 4° provides the most efficient lift/drag ratio, but in flight the propeller AOA of a fixed-pitch propeller varies—normally from 0° to 15°. This variation is caused by changes in the relative airstream, which in turn results from changes in aircraft speed. Thus, propeller AOA is the product of two motions: propeller rotation about its axis and its forward motion.
Propeller automatically keeps the blade maximum efficiency for most conditions ight. During takeoff, when maximum power required, the constant-speed propeller is at blade angle or pitch. The low blade angle small and efficient with respect to the relative wind. At the same time, it allows the propeller to handle a smaller mass of air per revolution. This light load allows the engine to turn at high rpm and to convert the maximum amount of fuel into heat energy in a given time. The high rpm also creates maximum thrust because, although the mass of air handled per revolution is small, the rpm and slipstream velocity are high, and with the low aircraft speed, there is maximum thrust.
After liftoff, as the speed of the aircraft increases, the constant-speed propeller automatically changes to a higher angle (or pitch). Again, the higher blade angle keeps the AOA small and efficient with respect to the relative wind. The higher blade angle increases the mass of air handled per revolution. This decreases the engine rpm, reducing fuel consumption and engine wear, and keeps thrust at a maximum.
After the takeoff climb is established in an aircraft having a controllable-pitch propeller, the pilot reduces the power output of the engine to climb power by first decreasing the manifold pressure and then increasing the blade angle to lower the rpm.
At cruising altitude, when the aircraft is in level flight and less power is required than is used in takeoff or climb, the pilot again reduces engine power by reducing the manifold pressure and then increasing the blade angle to decrease the rpm. Again, this provides a torque requirement to match the reduced engine power. Although the mass of air handled per revolution is greater, it is more than offset by a decrease in slipstream velocity and an increase in airspeed. The AOA is still small because the blade angle has been increased with an increase in airspeed.
what Degree is the slope of the propeller?
ReplyDelete